Studio pilota
Pilot study
Data collection, analysis and project development by O2UP srl
OBJECTIVE
To assess physiological responses to high-concentration, supplemental oxygen from the product UltraOxy in several types of subject and physical activity.
In addition, the study aimed to define practical means and methods for supplementation during sports activities.
INTRODUCTION
To date, few research studies in the scientific literature concern oxygen supplementation via portable devices.
In some studies, improved performance has been observed with administration of hyperoxic air via masks with stationary apparatus (Cardinale, 2018). The few studies that have been conducted have not investigated in depth the physiological response to and the methods of application of this substance.
Oxygen supplementation has often been associated with clinical therapy for neurological patients or patients with respiratory problems (White, 2013).
Sports performance is linked to improved competition results, as well as enhanced recovery capabilities in the short, medium and long term between competitions.
Enhanced performance and oxygen are often associated with the physiological changes that occur as a result of altitude training or due to methods that alter partial pressure through hyperbaric chambers (Bailey, 1997; Wilber, 2004).
Conversely, oxygen supplementation delivered through portable devices could become a new methodological approach in both sports and clinical practice, especially given the latest research carried out using stationary apparatus.
Few studies have provided supplementation guidelines for application times and number of inhalations. Some studies have only highlighted that physiological variations depend on the higher concentration of O2 inhaled or have shown improvements in terms of work performed (Heller, 1995), or that higher tissue concentrations lead to faster recovery, especially in intermittent activities (Nummela, 2002), although without studying cardiorespiratory and metabolic adjustments.
Thus, one interesting area of study is investigating physiological and cardiorespiratory responses, in addition to defining the means and methods for prescribing effective supplementation.
MATERIALS AND METHODS
The experimental protocol considered several types of sport and their related performance models. The following sports activities were selected for the study: Football/soccer, Endurance (running, cycling) and CrossFit.
Subjects:
The study population comprised male and female subjects, all in good physical condition at baseline (+1 year of training in the relevant sport), in ideal athletic condition (+3 training sessions/week), with no recent injuries.
The study included 20 athletes: 6 footballers, 6 CrossFitters (3 men and 3 women) and 8 endurance athletes (6 men and 2 women).
The mean age was 30 ± 5 years. Mean height was 177.5 ± 7.3 cm and mean weight 75.2 ± 8.6 Kg.
Procedure:
All subjects took part in two tests involving the same type of exercise and the same procedure in each.
The subjects performed a test at BASELINE (T0) aimed at observing their physiological response to the exercise that was specific to their sport, with no external factors affecting their performance.
The subjects underwent retesting (Texp), involving an additional variable, 7 days after T0.
During the second test session, each subject in the study group was randomly assigned a supplement, under double-blind conditions (with neither the subjects nor the investigators knowing the type of supplement administered), of an inert, odourless gas, administered from a portable canister, containing either 21% O2 breathable air (Air) or pure breathable oxygen (UltraOxy).
The supplementation protocol involved 8 inhalations (by mouth only, nostrils closed) before starting the exercise and during all phases of recovery in the test protocol.
The following parameters were measured using the Cosmed® K5 portable metabolic testing device throughout all control and experimental tests: VO2 (oxygen uptake, ml/kg/min), VE (ventilation, L/min), RF (respiratory frequency 1/min), RQ (respiratory quotient), HR (heart rate) TAU (kinetics of fast aerobic component uptake), LA (blood lactate).
Blood lactate was measured using the Arkray® Lactate Pro 2 device and the rating of perceived exertion was measured on the Borg CR-10 RPE scale.
Experimental Protocol:
Football protocol:
-
(T0)
5 min standardised W-UP,
3x4 min of shuttle runs over 25+25 m in 10 sec with 20 sec REC,
1 min 30 sec of MACRO REC,
Blood lactate taken at end of set + RPE,
Blood lactate 5 min after end of test. -
(Texp)
same as T0 but with 8 x INHALATIONS, before and during REC break.
Endurance Protocol:
-
(T0)
Maximal ramp test to establish VO2 max and VT2 anaerobic threshold,
3x4 min running/cycling at speed/power of VT2 anaerobic threshold,
1 min 30 sec of MACRO REC,
Blood lactate taken at end of set + RPE,
Blood lactate 5 min after end of test. -
(Texp)
ssame as T0 but with 8 x INHALATIONS, before and during REC break.
CrossFit Protocol:
-
(T0)
3x3 min ON / 1 min 30 sec OFF of: 20/16 cal. assault bike 20/16 DB hang snatches + max rep burpees,
Blood lactate taken at end of set + RPE,
Blood lactate 5 min after end of test. -
(Texp)
same but as T0 with 8 x INHALATIONS, before and during REC break.
Statistical Analysis:
All parameters measured in each test session were analysed in Excel and JASP.
These were shown as a mean, with standard deviations calculated, and with any differences between the two conditions determined by T-test.
RESULTS
A total of 20 subjects were assessed in the study.
All 20 participants performed a test at BASELINE (T0), receiving no supplements and with the same exercise procedures as used in the subsequent test performed with supplementation of 21% O2 breathable air or pure breathable oxygen (UltraOxy).
The mean oxygen uptake in the tests was 40.7 ± 5.2 and 46.0 ± 6.3 ml/kg/min in the two BASELINE T0 tests, and 39.4 ± 5.1 and 46.2 ± 6.0 ml/kg/min in the two Texp tests (AIR and O2; 8 BREATHS).
The mean value of the cardiorespiratory and metabolic parameters in the BASELINE (T0), Texp (Air) and Texp (O2; 8 BREATHS) tests is shown in the table below.
No significant difference in the parameters was found between BASELINE (T0) and Texp (Air).
On comparison of BASELINE (T0) and Texp (8 BREATHS O2), the ventilation parameter RF (respiratory frequency) and TAU (respiratory system kinetics) were significantly different (both p < 0.01).
The mean total value for RF was 47.3 ± 10.1 (T0), and 43.6 ± 9.0 in Texp (O2, 8 BREATHS).
TAU was 49.8 ± 12.8 sec (T0) and 42.1 ± 12.7 sec in Texp (O2, 8 BREATHS).
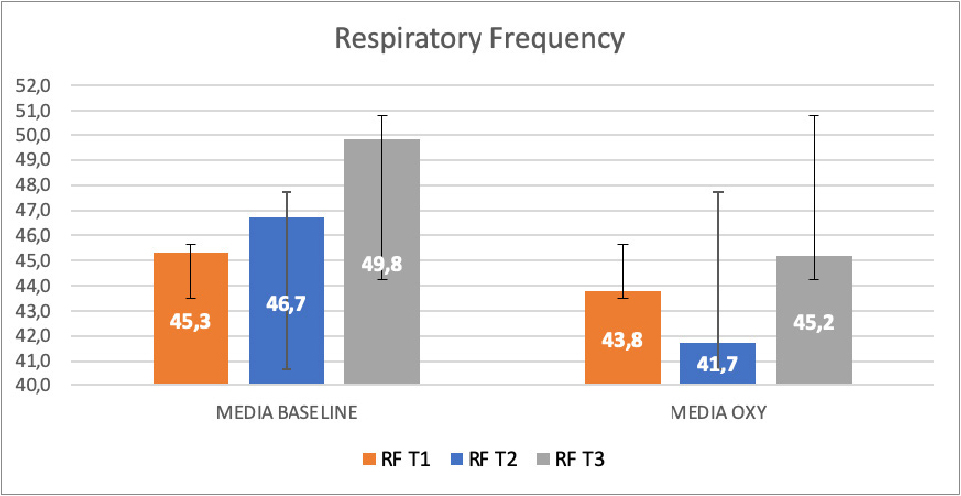
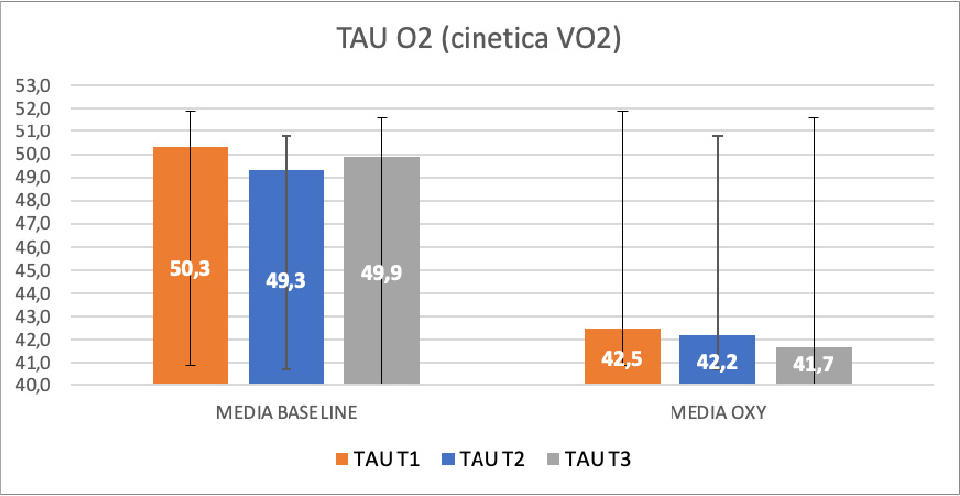
DISCUSSION
The aim of this study was to measure physiological responses to supplemental oxygen from a portable canister of UltraOxy in different types of subjects and sports. Additionally, the study aimed to determine whether a specific experimental protocol involving application of UltraOxy (8 breaths before and during exercise) was more effective than another. The adaptations were observed in the acute setting (within the exercise period) during different forms of physical activity.
It is important to mention that supplemental oxygen delivered by inhalation is not on the list of prohibited methods.
Some companies are currently investing in this area, despite a lack of extensive literature on the matter and that it is only recently that evidence has emerged that high-concentration oxygen supplements can enhance physical performance.
Recent studies have analysed physiological adaptations, in terms of changes in blood lactate, SpO2 and HR, after supplementation with hyper-oxygenated air. Rating of perceived exertion (RPE) and cognitive capacity are often investigated in addition to these.
Some studies have evaluated post-exercise responses in terms of oxidative and inflammatory stress. Most studies provide evidence, albeit without in-depth investigation of the cardiorespiratory component.
This pilot study is the first to measure cardiorespiratory responses to administration of breathable oxygen by inhalation.
In the acute setting, it is possible to observe that the uptake kinetics of the respiratory system and respiratory frequency in the study subjects are significantly lower in the Texp (O2; 8 BREATHS) tests than in the BASELINE T0 control.
Generally speaking, the body regulates respiratory frequency dynamically in order to maintain the balance between oxygen intake and carbon dioxide elimination, thereby ensuring the correct functioning of the body.
We know that this regulation responds to nervous or chemical stimuli.
Regulation primarily takes place through the nervous system, depending on the body’s requirements in terms of oxygen intake and carbon dioxide clearance.
The respiratory centre, located in the brain stem, plays a key role in this process.
Several factors affect how respiratory frequency is regulated:
-
Carbon dioxide (CO2) levels:
the main stimulus for respiration is the increase in blood CO2 levels. When CO2 increases, the body’s chemical receptors send signals to the respiratory centre to increase the respiratory frequency so that excess CO2 can be cleared. -
Oxygen (O2) levels:
the main stimulus is increased CO2, however blood oxygen levels can also affect respiration. Specifically, low oxygen levels can stimulate respiration. -
Blood pH:
changes in blood pH, mainly caused by the presence of CO2, can affect respiration. The body tries to maintain an acid-base balance and regulates breathing accordingly. -
Physical activity and metabolism:
exercise and metabolism affect the production of CO2 in the body. For example, respiratory frequency increases during physical activity to meet oxygen requirements and clear the CO2 produced during metabolism.
-
Nervous stimuli:
some nervous stimuli, including stress and anxiety, can affect respiratory frequency via the autonomic nervous system.
The hypothesis in this study is that when the test condition involved inhalation of air with hyper-concentrated oxygen (O2) at sufficient quantities and flow rates (8 BREATHS), oxygen intake was sufficient to modulate the peripheral nervous system’s control (linked to the chemical component) of the respiratory response.
Indeed, RF (respiratory frequency) was significantly lower (P < 0.01) at BASELINE T0 with 47.3 ± 10.1 (T0) than at Texp (O2; 8 BREATHS) with 43.6 ± 9.0.
It is important to note that only the 8 BREATHS test condition led to a minimum intake of hyper-oxygenated air that was sufficient to modify nerve-mediated respiratory responses, as this flow rate and quantity (approximately 30-40 sec. inhalation) are probably sufficiently effective.
The number of inhalations is in line with other studies that have reported performance improvements using similar methods (8 breaths / 30-45 sec).
This adaptive response to the decrease in breaths per minute was also associated with the presence of TAU, faster and significantly different VO2 response kinetics, still in the experimental group, than in the control (49.8 ± 12.8 sec BASELINE (T0) and 42.1 ± 12.7 sec in the Texp test (8 BREATHS O2); p < 0.01).
The TAU (time constant) for oxygen uptake is a parameter used to describe the dynamic response of the oxygen uptake system to input changes or stimuli.
Mathematically it represents the time it takes for a body’s oxygen uptake to reach 63.2% of the total variation in response to a disturbance, which in our study is associated with supplementation with air containing hyper-concentrated oxygen.
TAU is affected by several factors, including genetics, age, level of training, the health of the subject and the specificity of the exercise performed.
Two opposing hypotheses have been suggested regarding the control mechanism for VO2 kinetics.
One is that the rate of increase of VO2 at the start of the exercise is restricted by the ability to deliver oxygen to the active muscle.
The other hypothesis is that the ability to use oxygen in the muscle exercise acts as the limit factor on the rate of increase of VO2.
These hypotheses are still under discussion. It has certainly been shown in the sports and health fields that lower and faster TAUs may be associated with better performance (Dupont, 2005; Dupont, 2010), lower oxygen debt (EPOC) and, above all, faster recovery times, thanks to an accelerated adaptive response (Xu, 1999).
Examples of TAUs of some tested subjects:
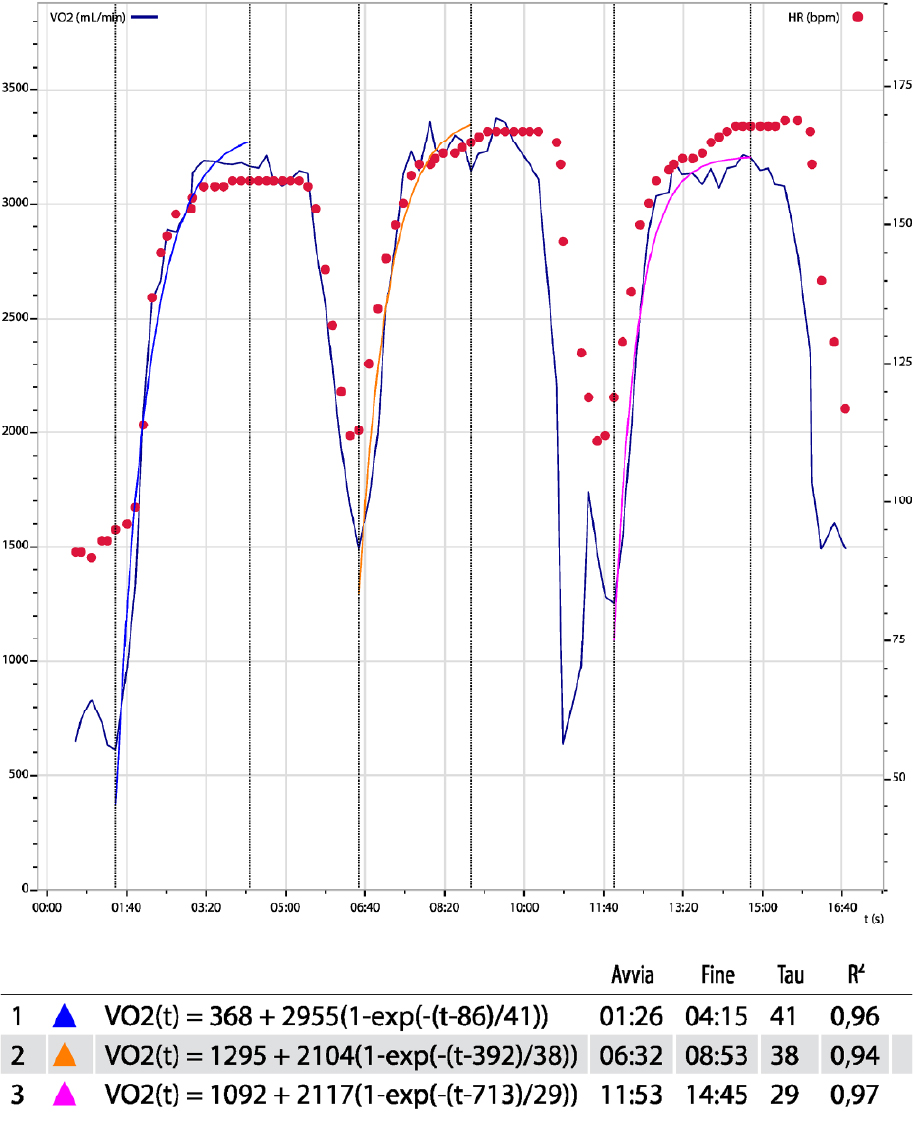
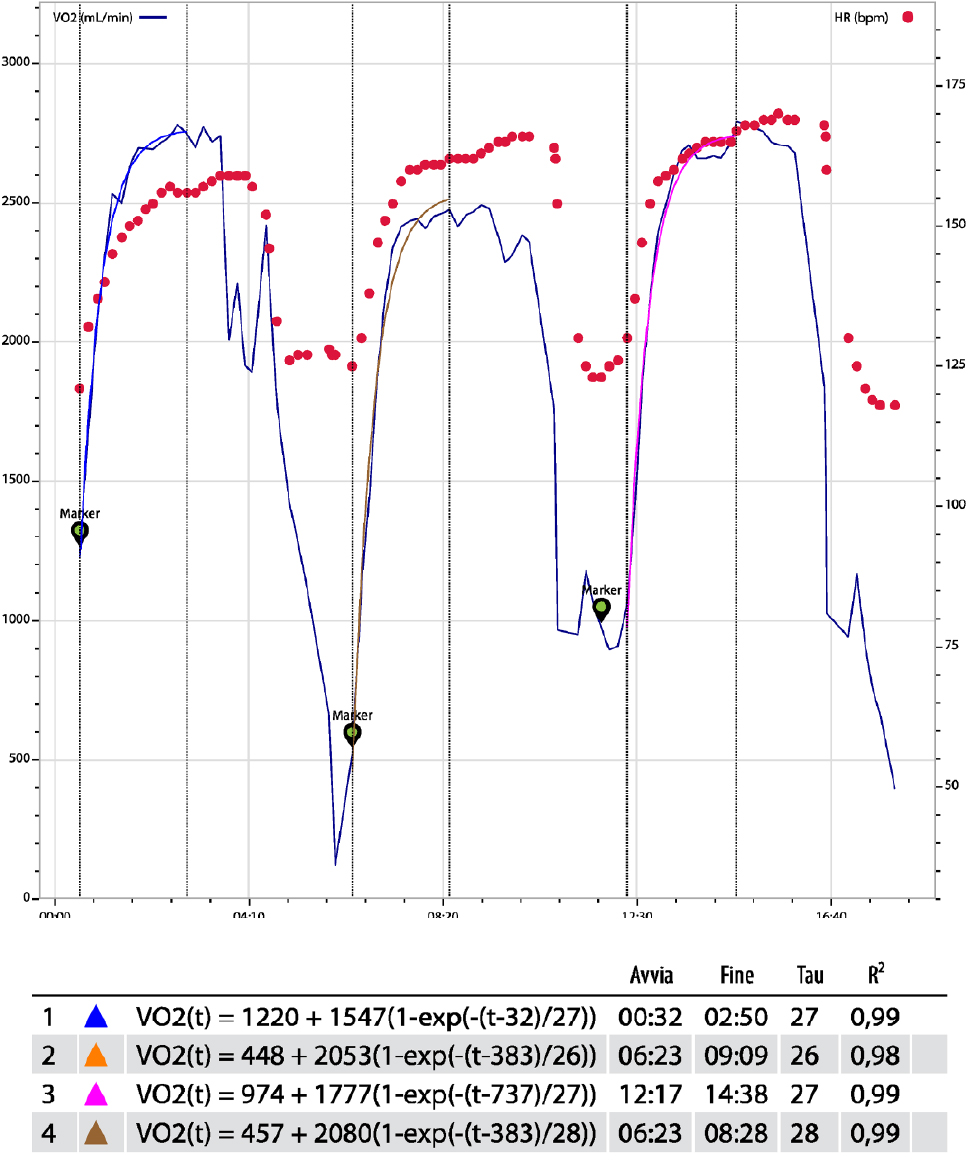
CONCLUSIONS
In practical terms, although this study was carried out on a limited sample size (n=20), it shows that supplementation with hyper-oxygenated air from a UltraOxy portable canister, led, in the 8 BREATHS test only, with a minimum application time of 30-45 sec, to lower respiratory frequencies in the subjects tested, which probably led to less work for the respiratory muscles, hence less fatigue (Mador, 1991).
Alongside the respiratory system’s improved capacity for work, in terms of both effectiveness and efficiency for muscle mechanics, faster VO2 TAUs were also observed.
This other adjustment in the acute setting would theoretically trigger metabolic recovery responses more rapidly at the peripheral and cellular level, as already observed in similar studies on the kinetics of the cardiorespiratory system (Healy, 2003).
In conclusion, the UltraOxy product has proven effective at improving the response of the mechanical respiratory pattern (RF), without altering cardiorespiratory parameters like VO2max or HR in controlled subjects.
Furthermore, supplementation with clearly defined application times (> 30 sec) would produce acute changes in the uptake kinetics of the aerobic system, limiting the initial oxygen debt and leading to a faster capacity for work and, consequently, recovery from the type of intermittent high-intensity exertion that can occur in such sports as CrossFit, football and certain endurance activities.
REFERENCES
Wyton, Lee (2018). Do portable recreational oxygen supplements share the same physiological benefits as otherforms of hyperoxic environments during moderate intensity cycling? University of Chichester, Sport and ExerciseDepartment.
CYR KIRK, Shannon; BILLAUT, François. Hyperoxia improves repeated sprint ability and the associated training loadin athletes. Frontiers in Sports and Active Living , 2022, 4: 817280
White, J., Dawson, B., Landers, G., Croft, K., & Peeling, P. (2013). Effect of supplemental oxygen on post exerciseinflammatory response and oxidative stress. European Journal of Applied Physiology, 113(4), 1059 1067.
MAEDA, Takafumi; YASUKOUCHI, Akira. Blood lactate disappearance during breathing hyperoxic gas after exercisein two different physical fitness groups on the workload fixed at 70% VO2max. Applied Human Science , 1997,16.6: 249 255.
CARDINALE, Daniele A.; EKBLOM, Björn. Hyperoxia for performance and training. Journal of sports sciences, 2018,36.13: 1515 1522.
Heller J. Diagnostika anaerobniho vykonua kapacity pomoci all out testu. Tel. vých. sport. mlád. 1995; 61:35 40.
BAILEY, Damian M.; DAVIES, Bruce. Physiological implications of altitude training for endurance performance atsea level: a review. British jo urnal of sports medicine , 1997, 31.3: 183 190.
Castillo RL, Salinas Y, Ramos D. Efectos biológicos de la exposición a distintas concentraciones de oxígeno: desde lahipoxia hipobárica al oxígeno hiperbárico [Biological effects due to exposure to different concentrations of oxygenfrom hypo to hyperoxemia]. Rev Med Chil. 2022 Oct;150(10):1351 1360. Spanish. doi: 10.4067/S003498872022001001351. PMID: 37358094.
WILBER, Randall L., et al. Effect of FIO2 on oxidative stress during interval training at moderate alti tude. Medicineand science in sports and exercise , 2004, 36.11: 1888 1894.
MADOR, Jeffery. Respiratory muscle fatigue and breathing pattern. Ches t , 1991, 100.5: 1430 1435
HEALY, Rhiannon D., et al. Relationship between VO2peak, VO2 Recovery Kinetics, and Muscle Function in OlderAdults. Gerontolog y, 2023, 69.11: 1278 1283
DUPONT, Grégory, et al. Relationship between oxygen uptake kinetics and performance in repeated runningsprints. European journal of applied physiolog y , 2005, 95.1: 27 34
DUPONT, Gr egory, et al. Faster oxygen uptake kinetics during recovery is related to better repeated sprintingability. European Journal of Applied Physiolog y , 2010, 110: 627 634
XU, Fan; RHODES, Edward C. Oxygen uptake kinetics during exercise. Sports medicine , 1999, 27: 313 327.
SUCHÝ, J.; HELLER, J.; BUNC, V. THE EFFECT OF INHALING CONCENTRATED OXYGEN ON PERFORMANCE DURINGREPEATED ANAEROBIC EXERCISE. Biology of Sport, 2010, 27.3
Heller J. Diagnostika anaerobniho vykonua kapacity pomoci all out testu. Tel. vých. sport. mlád. 1995;61:35 40.
Nummela A., Hamalainen I., Rusko H. Effect of hyperoxia on metabolic response and recovery in intermittentexercise. Scand. J. Med. Sci. Sports. 2002;12:309 315